The Philippines was under attack by a killer.
The entire world knew about this killer. Everyone despised it. The evidence of its misdoings was undeniable. Yet it was impossible to bring this killer to justice. After all, it wasn’t human.
It was dengue fever.
Dengue fever is a sometimes fatal disease caused by the dengue virus and spread by mosquitoes2. In places like the Philippines, it can be a leading cause of death2.
But there was promise. A new vaccine, creatively named “Dengvaxia,” had the potential to prevent dengue fever.
The Philippines went all-in on a vaccination campaign. Eventually, it reached over a hundred thousand Filipino children3. Everything was going well.
Until it wasn’t.
The human body fights off disease using a variety of mechanisms. One of the important ones is the antibody, which marks germs for destruction and makes it hard for the germs to wreak havoc4. After catching the disease for the first time or being vaccinated, the body prepares itself to make antibodies more efficiently next time. Ordinarily, this would be good — but, as it turns out, dengue virus uses antibodies to transport itself throughout the body, in a phenomenon known as antibody-dependent enhancement5. A study released in November 2017 showed that Dengvaxia can also stimulate antibody-dependent enhancement3.
In other words: In people who hadn’t caught dengue fever before, the vaccine could actually make the disease worse.
And then the world burned!
Well, it didn’t literally burn, but things got really bad. First of all, the vast majority of those 100 000 kids were now at a higher risk for dengue complications. And while that’s bad enough, things got worse — because of fear. The scandal gained a lot of publicity, leading to some very concerned — and, in many cases, angry — parents. These parents decided to simply not vaccinate their kids at all. This is a recipe for disaster. That disaster struck in 2019, when a measles outbreak hit. The unvaccinated kids were barely protected against the disease. It spread like wildfire throughout the Philippines, infecting 23 563 people and killing 338 of them6.
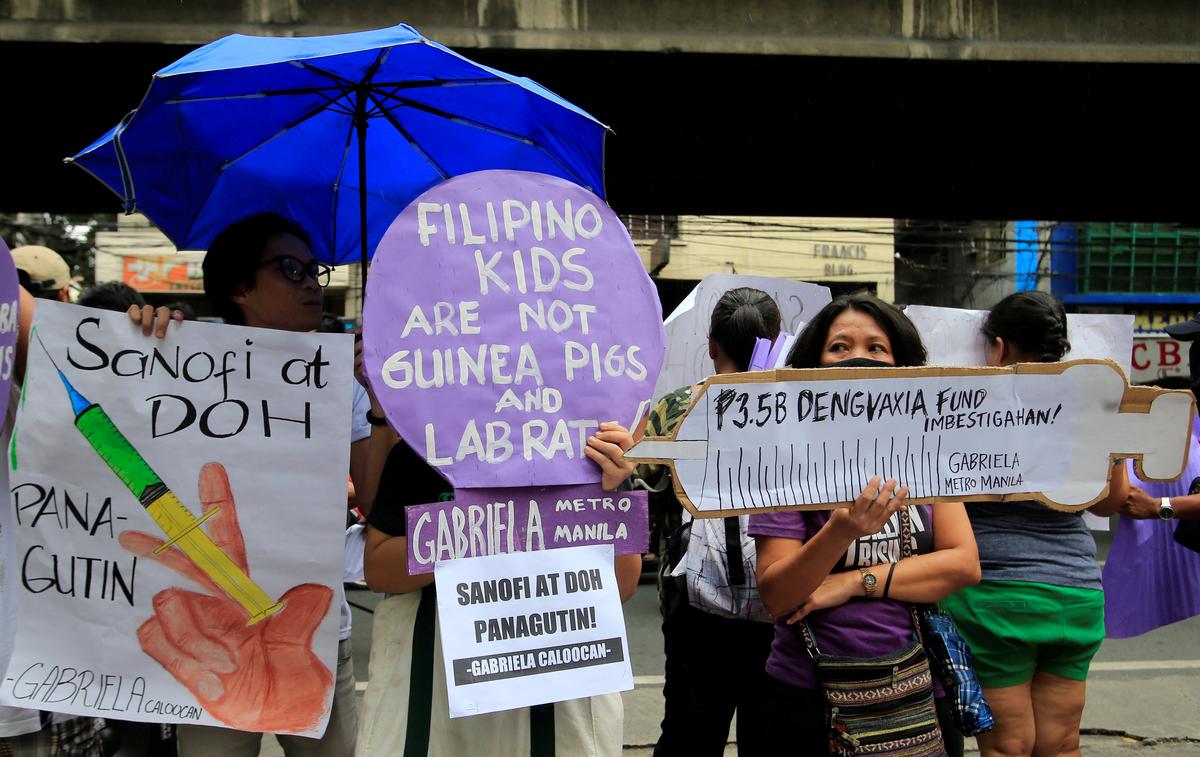
Shortly after a study showing the issues with Dengvaxia, protestors demonstrate in front of the Philippine Department of Health.
Credit (as per the Reuters Brand Attribution Guidelines): REUTERS/Romeo Ranoco
And the worst part? Based on the original clinical trial data for Dengvaxia, one scientist suspected that the vaccine might have disastrous effects — but that data wasn’t detailed enough to actually prove his suspicions3. Had the data been more thorough, this entire chain of unfortunate events could have been avoided.
The moral of the story is that we can’t get rid of clinical trials. We can’t sacrifice their accuracy. People’s lives are at stake. When we make clinical trials faster, we need to make sure they’re still accurate.